Scientists explore the creation of artificial organelles
Artificial organelles generated from Exosome fusion can function as energy reserves in the damaged
tissues
The human body is made of numerous different types of cells, which have small compartments known as organelles to
perform complex biochemical reactions. These compartments have multiple enzymes that work together to execute
important cellular functions. Researchers at the Center for Soft and Living Matter within the Institute for
Basic Science (IBS, South Korea) have successfully mimicked these nano spatial compartments to create
‘artificial mitochondria’ in the latest research published in Nature Catalysis as a cover article. They
state the technology can be
used to construct artificial organelles that can supply ATP or other useful molecules to cells in damaged or
diseased tissues.
This was achieved through reprogramming of ‘exosomes’, which are small vesicles (diameter ~120 nm) that cells use
for intercellular signaling. The researchers carried out the experiments using microfluidic droplet reactors,
which generated small droplets that were of similar size as typical cells. (diameter ~10 μm) The researchers
first aimed to facilitate controlled fusion of these exosomes within the droplets while preventing unwanted
fusions.
They accomplished this by tailoring the exosome surfaces with molecules called catechol, which is a chelating
agent that forms complexes with metal ions. This was in turn done by attaching the catechol onto antibodies that
target specific cell markers, such as CD9. The complex-forming property of catechol allows them to drive fusions
between exosomes when they are mixed with metal ions such as Fe3+. The membrane fusion occurs when
the catechols
on the surfaces bind to the iron and bring the vesicles to close proximity to one another.
Researchers first tested the effectiveness of this system by loading one type of exosomes with
calcein-Co2+ and
another with EDTA. When the two vesicles fuse and the contents are mixed, EDTA grabs the Co2+ away
from calcein,
which then allows the latter to fluoresce. The team realized they were successful upon the detection of the
fluorescence signal, and the fusion was further confirmed by the doubling of the measured exosome diameter.
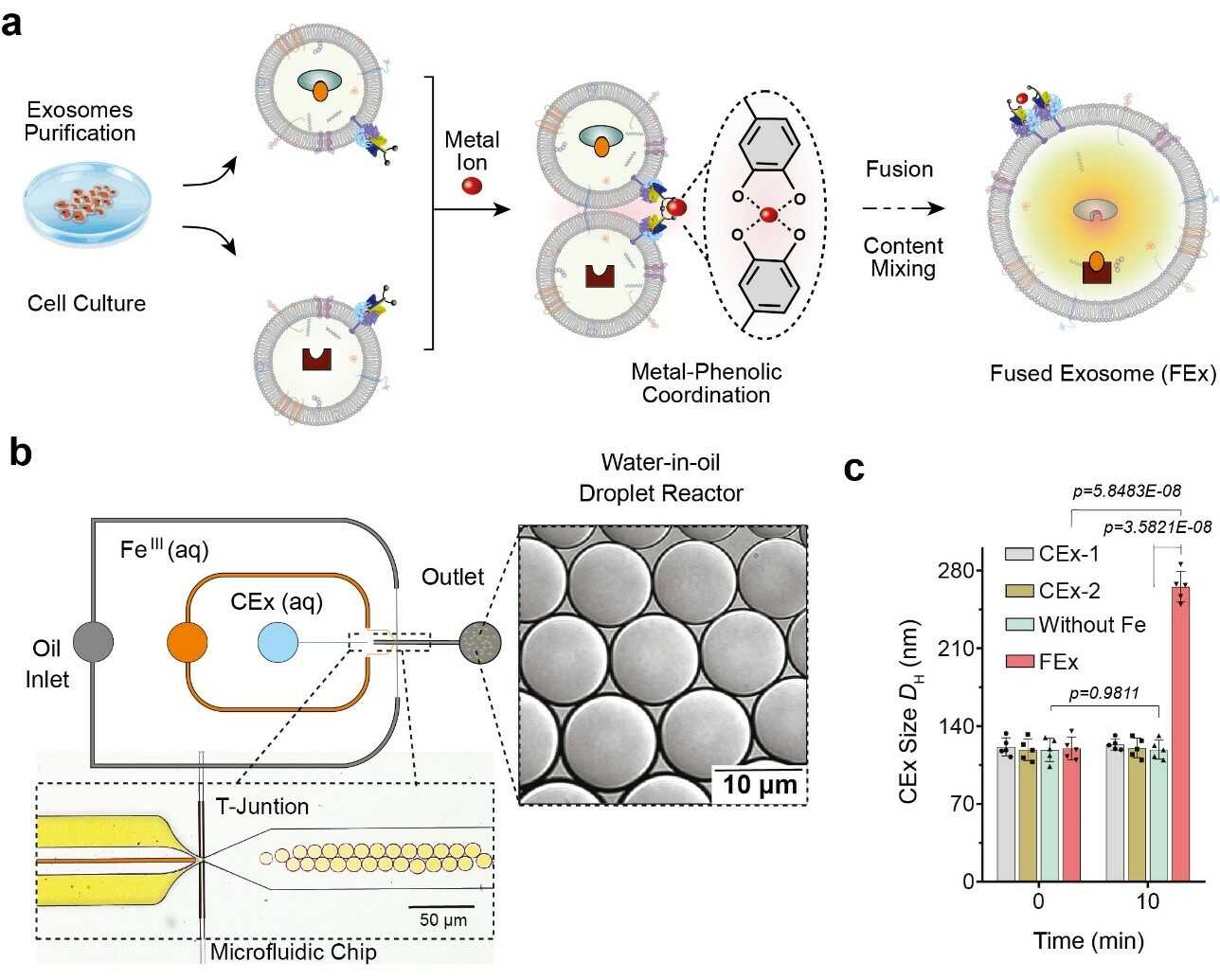
Figure
1. (a)Fe3+ ion triggers membrane fusion in exosomes tailored with catechol (CEx). The
formation of a metal-catechol complex brings the exosomes into close proximity and enables fusion. Different
reactants in each exosome are then encapsulated together, promoting mixing and triggering reactions inside the
fused exosomes (FEx). (b) The microfluidic device designed for generating water-in-oil droplet reactors
at the flow-focusing junction. The two aqueous phases (CEx and metal salt) meet and are delivered into the oil
stream (1% surfactant PFPE-PEG in FC-40) to break the liquid into droplets. (c) Average size of unfused
exosomes (CEx-1: 121 ± 8 nm, CEx-2: 123 ± 5 nm) and fused exosomes (FEx: 265 ± 14 nm).
These customized exosomes were then preloaded with different reactants and enzymes, which turned them into
biomimetic nano factories. This allows them to produce high-value biomolecules by performing desired
biocatalytic transformations in a spatially confined manner which is not possible using conventional laboratory
test tubes. The team demonstrated this multienzyme biocatalytic cascade function by encapsulating glucose
oxidase (GOx) and horseradish peroxidase (HRP) inside the exosomes. The GOx first converts
glucose into gluconic acid and hydrogen peroxide. The HRP in turn uses the hydrogen peroxide generated in the
first reaction to oxidize Amplex Red to a fluorescent product, resorufin. The researchers were even able to take
a step further by adding a third enzyme, galactosidase which converts lactose into glucose, into the mix.
Next, the researchers wanted to know exactly how well these mini reactors can be uptaken and internalized by the
cells. The cells derived from human breast tissues were fed with fused exosome nanoreactors, and their
internalization over the next 48 hours was observed using various markers and a confocal laser scanning
microscope (CLSM). It was found that cells were able to uptake these customized exosomes primarily through
endocytosis, along with multiple other mechanisms. They further tested the beforementioned GOx-HRP
two enzyme system in the cells, and it was found that the fused exosomes were able to successfully manufacture
fluorescent products even while being inside the cells.
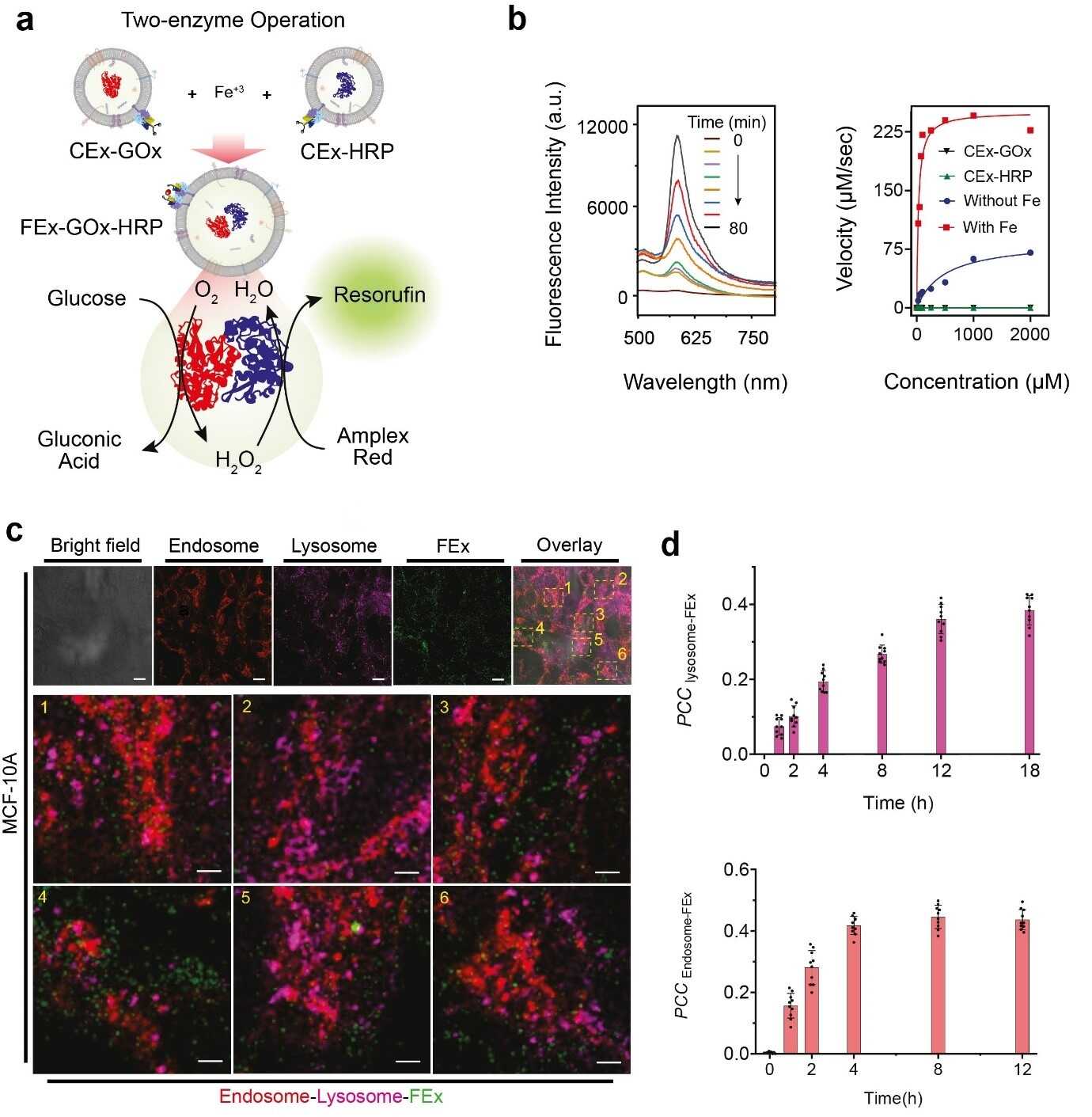
Figure
2. (a)Schematic representation of a two-enzyme GOx/HRP CEx fusing into FEx. The catalytic
reaction was initiated by adding glucose, generating H2O2 by aerobic oxidation, which was
then used to oxidize Amplex Red to generate resorufin. (b) Representative fluorescence spectra of
resorufin generated by biocatalysis in fused exosomes (FEx-GOx-HRP) at different time
points. Reaction rate for the nanoreactor vs glucose concentration to calculate kinetic constants by fitting the
Michaelis-Menten equation. (c) Stability and integrity of FEx-GOx-HRP after
cellular integration after 48 h. Endosomes (EEA1, red) lysosomes (LAMP1, magenta), and FEx (green)
are shown. (d) Plot of Pearson correlation coefficient (PCCLysosome-FEx) values over
time for magenta (lysosome) and green (FEx) fluorescence, and for red (Endosome) and green
(FEx) fluorescence.
Armed with this knowledge, the team sought to create functional artificial mitochondria that are capable of
producing energy inside the cells. To achieve this, ATP synthase and bo3 oxidase were reconstituted into the
earlier exosomes containing GOx and HRP, respectively. These exosomes were in turn fused to create
nanoreactors that can produce ATP using glucose and dithiothreitol (DTT). It was found that the fused exosomes
were capable of penetrating deep into the core part of a solid spheroid tissue and produce ATP in its hypoxic
environment. The activities of these simple organelles were accompanied by marked reduction of reactive oxygen
species (ROS) generation. In contrast, free enzymes were unable to penetrate inside these tightly packed
spheroids of cells.
“Taken together, our results highlight the potential of these exosomes as nanoreactors in regulating the
metabolic activity of cells inside spheroids, and in attenuating cell damage due to hypoxia,” notes CHO
Yoon-Kyoung, the corresponding author of the study. It is hoped that further research into such artificial
organelles will present a new paradigm in various fields such as disease diagnosis and treatment, biotechnology,
medicine, and the environment.
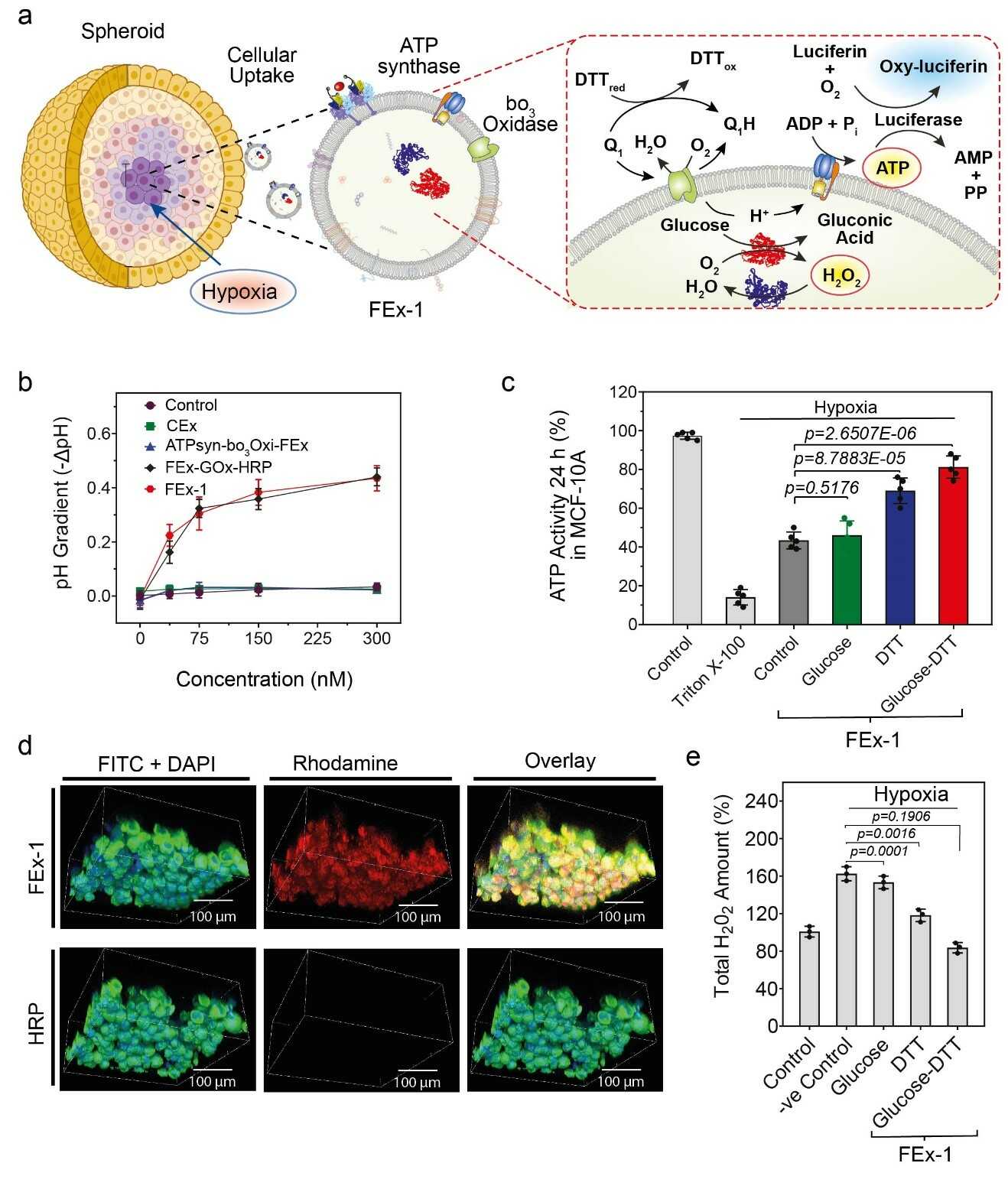
Figure
3. (a) Assembly of artificial organelles for energy generation. ATP synthase was reconstituted into CEx-GOx and bo3 oxidase was reconstituted into CEx-HRP. The two CEx were fused and were able to penetrate deep into spheroids. (b) Decrease in pH (-ΔpH) vs. glucose concentrations for different exosomes. Fused exosomes show a greater degree of pH decrease. (c) Changes in ATP production after FEx-1 uptake under different conditions. (d) Artificial organelles were able to penetrate deep into the spheroid. The top row shows FEx-1 (labeled red with rhodamine B), while the bottom row shows free HRP enzyme inside spheroids. (e) Overall ROS levels decreased on subjecting FEx-1 under glucose and DTT.
Notes for editors
- References
Sumit Kumar, Mamata Karmacharya, Issac J. Michael, Yongjun Choi, Junyoung Kim, InUn Kim, Yoon-Kyoung Cho. Programmed exosome fusion for energy generation in living cells. Nature Catalysis 2021.
- Media Contact
For further information or to request media assistance, please contact Yoon-Kyoung Cho(yoonkyoung.cho@gmail.com) at the Center for Soft and Living Matter, Institute for Basic Science (IBS) or William I. Suh at the IBS Communications Team (willisuh@ibs.re.kr).
- About the Institute for Basic Science (IBS)
IBS was founded in 2011 by the government of the Republic of Korea with the sole purpose of driving forward the development of basic science in South Korea. IBS has 33 research centers as of July 2021. There are ten physics, three mathematics, six chemistry, eight life science, one Earth science, and five interdisciplinary research centers.